Chapter 12: Intermolecular Attractive Forces
On several occasions up to this point I have used the term 'intermolecular
attractive forces'. I first used it when we looked at the animation of the container
of gas as it was cooled. (See the phase transition animation
at the particulate level.) Recall in this animation a container of gas particles
was cooled. As the temperature of a collection of particles was lower we observed
the particles slowing down. At the lower velocities colliding particles appeared
to stick together forming groups of particles. As the temperature continued
to drop the number of particles in these groups increased. Eventually the groups
of particles are of sufficient size that they fall to the bottom of the container
as a result of force of gravity, forming a liquid. As the tmperature continues
to drop the particles become more ordered, and their translational energy drops
to a very small value and a solid forms. Condensation occurs when the intermolecular
attraction between a pair of particles exceeds the kinetic energy of the collision.
The 'stickyness' exhibited by particles at the lower temperatures, which
result in the formation of liquids and eventually solids is due to intermolecular
attractive forces. Intermolecular means between molecules. Intramolecular means
between atoms. Intramolecular forces are what we call covalent bonds and are
very strong (100 - 1000 kJ/mol) (see also
Table 9.2 in Silberberg). Intermolecular forces are between molecules and are
weak (0.1 - 40 kJ/mol). Intermolecular forces are less directional compared
to covalent bonds and operate over a longer range compared to covalent bonds.
It is intermolecular forces which explain the formation of liquids and solids
in covalent compounds. Intermolecular attractive forces are electrostatic in
nature.
Intermolecular forces are classified into the following categories;
* ion-dipole (we will discuss when we cover Chapter 13)
* dipole-dipole
* induced dipole-induced dipole (London dispersion forces)
* hydrogen-bonding
To determine which type(s) of intermolecular attractive forces occur for
a particular substance the most important characteristic to determine for the
substance is its polarity. A polar molecule possess a permanent dipole moment
(see pages 384 - 386 in Silberberg) as a result of its molecular shape and from
the unequal sharing of electrons in chemical bonds which produces the separation
of charge which produces the dipole.
To recognize a polar substance (you have to do this on the review problem
set) you must draw the Lewis structure (see pages 361 - 371 in Silberberg, CHEM
1314 lecture notes). After drawing a Lewis structure look at the central
atom.
Rules for predicting whether a molecule is polar (has a permanent dipole)
or is nonpolar
-
If the central atom has one or more unshared, nonbonding, pairs of electrons
the molecule is most likely polar. Examples include; NH3, H2O,
SO2
-
If the central atom has no unshared, or nonbonding, pairs of electrons
and nonidentical terminal atoms the molecule is polar.
-
If the central atom has has no unshared, or nonbonding, pairs of electrons
and the terminal atoms are identical the molecule is nonpolar.
A simple example is HCl. The Lewis structure for HCl is;

The pair of electrons in the covalent bond between hydrogen and chlorine is
unequally shared due to the difference in electronegativity between hydrogen
and chlorine. Chlorine has a greater electronegativity compared to hydrogen
and as a result the electrons in the covalent bond spend a greater proportion
of the time closer to the chlorine nucleus. So for chlorine the electrons in
the covalent bond spend more time nearer its nucleus producing a small partial
negative charge in the molecule. Since the hydrogen atom 'sees' the electron
in the covalent bond less frequently it has a partial positive charge. This
permanent separation of some small amount of charge on the HCl molecule produces
a permanent dipole. Here is a picture of the charge on a space-filling model
of the HCl molecule;
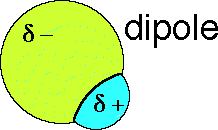
A collections of HCl molecules will align themselves such that the negative
end of one HCl molecule is attracted to the positive end of an adjacent HCl
molecule.
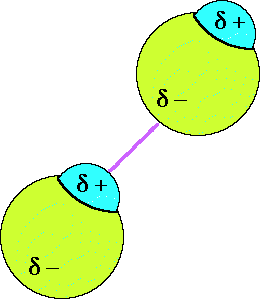
The ion-dipole force results from the attraction of an ion of positive or
negative charge and the oppositely charged end of the dipole on the polar molecule.
The strength of the attraction depends on the magnitude of the charge on the
ion, the magnitude of the dipole moment and the distance between center of the
ion and the midpoint of the polar molecule. Ion-dipole forces are important
for ionic compounds which dissolve in water.
Dipole-dipole forces of attraction occur between polar molecules. This type
of attractive intermolecular force occurs between the polar molecules of a pure
substance, such as HCl, or between two different polar molecules. Here is an
animation depicting the attraction when two HCl
molecule approach each other. The attraction arises because of the permanent
dipole in the polar molecule. Dipole-dipole attractions are relatively weak
compared to ion-ion attractions, because the charges on polar molecules are
generally quite small. In liquids the molecules are free to move relative to
each other, but will do so such that sometimes orientations of adjacent molecules
are attractive and sometimes they are repulsive. The overall average effect
is attractive.
London dispersion forces occur between atoms
or molecules of nonpolar substances. Monoatomic atoms (noble gases), diatomic
molecules (H2, N2, O2, F2, Cl2)
and nonpolar compounds (CH4, CCl4, BF3, BeH2,
etc.) are all characterized by a symmetric sharing of electrons in the atom
or molecule. These compounds do not have permanent dipoles as occur in heteronuclear
diatomic molecules (HCl, HBr, HI, etc) and polar compounds (SO2,
H2S, NCl3, etc).
In class we saw an animation of a collection of monoatomic neutral atoms.
If we take "snapshots" of the electron distribution we would generally see a
symmetric distribution of the electron density. However, occasionally we see,
but not very often, an unequal sharing of the electrons. A "snapshot" an instant
later would reveal a return to an equal distribution of the electrons. Every
once and a while we observe that the electrons are unequally distributed around
the nuclei, when this occurs there is a very small charge separation created
which gives rise to an instantaneous dipole.
Another atom near this instantaneous dipole will also be effected causing
a shift of its electron distribution resulting in a small dipole around it.
When this occurs, even for an instant there is a small attraction between the
two molecules. The strength of the London dispersion forces depends on how easily
the electron cloud is distorted or polarized.
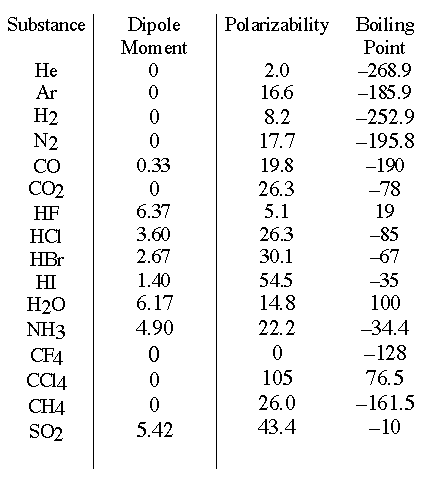
The larger the molecule the further the electrons are from the nucleus and
the easier the electron cloud can be polarized. So the magnitude of the dispersion
forces increases with increasing molecular size. Note: dispersion forces operate
in all molecules whether they are polar or nonpolar.
It has been shown (not in class) that the force of attraction between two
nonpolar molecules is inversely proportional to the seventh power of the distance
and directly proportional to a property of each molecule called polarizability.
Polarizability of an atom or a molecule is a measure of the ease with which
the electrons and nuclei can be displaced from their average positions. When
the electrons occupy a large volume of space, which occurs in an atom or molecule
with many electrons, the polarizability of the substance is large. The units
on polarizability are the units of volume, m3.
When the polarizability is large for a particular atom or molecule the magnitude
of the instantaneous dipole can be large with the result producing a stronger
attraction between particles.
The electrons which are the most easily displaced in an atom or molecule
are the valence electrons, these are the furthest from the nucleus. So valence
electrons make the greatest contribution to the polarizability. The force acting
on the valence electrons depends on their distance from the nucleus and on the
core charge. For any group in the periodic table the core charge remains constant,
so we expect polarizability to increase as the atomic size increases. So the
polarizability of HI is greater than the polarizability of HF.
In molecules with large numbers of atoms the polarizability will be larger
compared to smaller molecules. The polarizability of a molecule increases with
both increasing size and increasing numbers of atoms in the molecule. So we
expect the magnitude of the instantaneous dipoles, and therefore the strength
of the London forces, to be greater the greater the number of atoms in a molecule
and the larger the atoms.
The polarizability of N2 is greater than H2, and that of CCl4 greater than
CH4, and CO2 greater than that of CO. The strength of the intermolecular attractive
forces is reflected in the boiling points of the substances.
Hydrogen-Bonding
Two contributions to the intermolecular attractions between covalent molecules;
1) dipole-dipole forces (present only when the molecule is polar) and, 2) London
forces (present between all molecules and are particularly important for large
molecules.) London forces are often stronger than the dipole-dipole forces between
polar molecules. It is rare that dipole-dipole forces will dominate the properties
of a molecule unless the dipole-dipole forces are particularly strong. This
occurs for hydrogen-bonded systems.
-
Most solids expand up to 10 % of their volume when they melt. Water expands
by the same amount when it freezes!
-
Most solids are more dense than the liquid phase. However, ice has a density
of 0.917 g/cm3 while liquid water has a density of 0.998 g/cm3.
-
Water has a melting point which is 100 degrees C higher than expected
for its group of hydides.
-
Water has a boiling point 200 degrees C higher than expected for its group
of hydrides.
-
Water has the highest surface tension of any liquid except mercury.
-
Water is an excellent solvent, dissolving many ionic compounds which are
insoluble in other compounds.
-
Water has a high heat capacity.
All of these properties can be understood in terms of the intermolecular attractive
forces which exist between water molecules. The particular intermolecular attractive
force is called hydrogen-bonding. Hydrogen bonding is another intermolecular
force, which is stronger than London and dipole-dipole forces. Hydrogen bonding
forces occurs in a particularly special group of polar compounds. These compounds
are characterized by the X-H bond, where X can be O, N, or F. Examples of compounds
that exhibit hydrogen bonding forces are H2O, NH3 and
HF. Oxygen, nitrogen and fluorine are small strongly electronegative atoms.
In a covalent bond with hydrogen these atoms attract the pair of electrons giving
rise to a partial positive charge on the hydrogen atom. This partial positive
charge on the hydrogen atom is very interested in any negative charge in another
adjacent molecule that comes close to it. So when another polar molecule which
contains an atom such as O, N, F, Cl or S come near an X-H bond a hydrogen bond
can form.
So what would a hydrogen-bonding interaction look like when two or three molecules
of water were close to each other? To do this lets begin with just a lewis structure
for water. Draw the Lewis structure for water. When you are finished look at
my drawing.
Bulk Properties of Liquids
As an introduction to the need to define and discuss intermolecular attractive
forces we began our discussion of the bulk properties of gases, liquids and
solids. Some of these are discussed in Section 12.4 on pages 440 - 447 in Silberberg.
We talked about the following bulk properties;
* molar volume
* compressibility
* thermal expansion
* viscosity
* surface tension
Molar volumes of gases are much larger compared to the molar volume of liquids
and solids. For gases, assuming ideal behavior, the molar volume is 22,400 mLs
at 0 degrees C and 1 atmosphere. For liquids and solids the molar volume ranges
between 10 and 100 mLs at room temperature and pressure. Molar volume is inversely
proportional to the density of the substance.
Compressibility is a measure of the extent to which the volume of a given
amount of substance will shrink when it is squeezed. Liquids and solids are
nearly incompressible, while gases are highly compressible. The high compressibility
of gases is a result of the large amount of empty space which exists between
gas particles. In liquids and solids very little free space is present between
particles. Compressing liquids or solids, even at high pressures, results in
very small changes in volume.
Thermal expansion is the increase in volume of a substance with increasing
temperature. For ideal gases the coefficient of thermal expansion is 1/273 at
0 degrees C. A temperature increase of 1 degrees C changes the volume of a gas
by 0.366 %. At 25 degrees C the volume of 1 g of water is 1.00296 mL, at 26
degrees C the volume is 1.00323 or a change of about 0.026 %.
Viscosity measures the resistance to flow for a liquid. The higher the viscosity
the slower a liquid will flow. From a atomic view viscosity can be understood
in terms of the ability of particles to slide over and past each other. If a
fluid has a high viscosity particles "stick" to each other and do not slide
past each other easily. One wonders what causes this stickiness in the liquid
phase.
Surface tension measures how much energy is required to move a particle from
the middle of a fluid to the surface. The easier this can occur the lower the
surface tension. Liquids under the influence of gravity and on a surface for
which the adhesive forces are small, e.g. water on wax paper, will cause the
liquid to "bead-up". In an environment where the gravity is low or absent fluids
will form a spherical shape as this shape minimizes the surface area. Water
has a comparatively high surface tension. It is difficult to move a liquid from
within the liquid to the surface. One wonders why it is difficult to move a
molecule of water from within the middle of the liquid to the surface. Water
molecules must "stick" to each other.