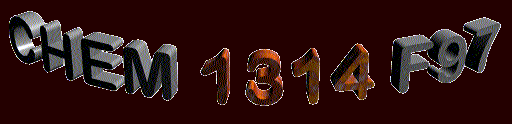
VSEPR is a useful and organized summary of experimental
results, and allows us to predict the 3-dimensional geometry of a
large number of covalent compounds and polyatomic anions. At this
level it would also be nice to try to understand how we might
view these geometries in terms of the orbitals that are used for
bonding by the atoms in these molecules. Two theories have
evolved from quantum mechanics which assist the chemists in
describing the experimental observations in terms of the atomic
or molecular orbitals in a compound. One is Valence Bond
Theory (pages 309 - 111) and the other is Molecular
Orbital Theory (pages 324 - 335). We will focus our attention
on VB Theory. We will not discuss the bonding in these molecules
in terms of MO theory.
VB theory is a way of describing the electron pair bonds that
occur in covalent compounds. This theory has too important
underlying assumptions.
An orbital on one atom comes to occupy a portion of
the same region of space as an orbital on the other. When
this occurs the two orbitals are said to overlap.
The total number of electrons in both orbitals is no
more than two.
When an orbital, occupied by a valence electron, on an atom
overlaps with an orbital containing a valance electron on another
atom the electrons in the orbitals begin to move about both
atoms. The attraction that each electron experiences from both
nuclei pull the atoms together. The strength of the bond depends
on the amount of overlap; the greater the overlap of the orbitals
the stronger the bond. Also remember the two overlaping orbitals
can not hold more than two electrons and their spins must be
opposite.
In the formation of the chemical bond in dihydrogen, H2,
two atoms of hydrogen, each with a single valence electron in a
1s orbital approach each other. For hydrogen the valence electron
is in a 1s orbital which has spherical symmetry. As they get
close the two 1s orbitals overlap with each other forming a
chemical bond. Here is a QuickTime movie
of the chemical bond in H2.
Helium atoms will not bond to each other as their 1s orbitals
are filled, overlap between two filled 1s orbitals would place
four electons in the same region of space. According to our model
only two electrons can occupy the same region of space. So He2
does not exist.
We can understand the bonding in HF using our VB description
also(Valence Bond Model). The 1s
electron on hydrogen overlaps with the singly occupied 2p orbital
on fluorine. Here is a QuickTime movie of
the chemical bond in HF. The movie shows the electron
configuration for a fluorine and for a hydrogen atom. The
fluorine atom has seven valence electrons with an unpaired
electron in one of its 2p orbitals. The hydrogen atom
has one valence electron in its 1s orbital. When the 2p
orbital on the fluorine atom overlaps with the 1s
orbital on hydrogen a covalent bond forms. An extension of this
approach is found in F2 where the half-filled 2p
orbital on each fluorine atom overlaps to form the covalent bond.
In water the oxygen atom has six valence electrons. Two of
the 2p orbital on the oxygen atom are half-filled. These
two half-filled orbital could bond to two hydrogen atoms. Here is
a QuickTime movie of the chemical bond
in H2O. The movie shows the electron configuration for
an oxygen atom and for the hydrogen atoms. The oxygen atom has
six valence electrons with an unpaired electron in two of its 2p
orbitals. The hydrogen atom has one valence electron in its 1s
orbital. When the two 2p orbitals on the oxygen atom
overlaps with the 1s orbital on the hydrogen atoms
forming two covalent bonds. The interesting observation is the
bond angle we would expect from our Valence Bond Model is 90
degrees, but the experimental observation is a bond angle of 105
degrees. To explain the experimental bond angle we will need use
different orbitals on the oxygen atom.
Another conflict with the Valence Bond Model is found with
ammonia, NH3. Here is a QuickTime
movie of the chemical bond in NH3. The movie shows
the electron configuration for a nitrogen atom and for the
hydrogen atoms. The nitrogen atom has five valence electrons with
an unpaired electron in each of its 2p orbitals. The
hydrogen atom has one valence electron in its 1s
orbital. When the three 2p orbitals on the nitrogen atom
overlaps with the 1s orbital on the hydrogen atoms
forming three covalent bonds. The interesting observation is the
bond angle we would expect from our Valence Bond Model is 90
degrees, but the experimental observation is a bond angle of
107.5 degrees. To explain the experimental bond angle we will
need use different orbitals on the nitrogen atom.
Finally a new question arises when we look at the electron
configuration for carbon. It has four valence electrons, two in
the 2s orbital and two electrons in the 2p
orbitals. Experimentally the simplest compound containing carbon
and hydrogen is methane, CH4. All four of the C-H
bonds are identical in length and all the bond angles are the
same. How can we understand this experimental fact with our VB
model? The answer is--we can not unless we modify the model. The
way we modify the model is to promote a 2s electron into
the empty 2p orbital. This promotion would require energy, which
is more than made up for by the formation of two additional
bonds. With this new 'promoted' electron arrangement on the
carbon atom three 1s orbitals on hydrogen would interact with the
three singly occupied 2p orbitals and one 1s orbital on hydrogen
would interact with the singly occupied 2s orbital. We would
expect two different kinds of bonds. Experimentally we only
observe one kind of C-H bond, that is, all are the same. To
explain this, VB theory postulates that after promoting the 2s
electron into the empty 2p orbital a reorganization of the atomic
orbitals on carbon occurs to produce four identical orbitals.
Here is a QuickTime movie of the promotion and mixing of the
atomic orbitals on carbon.
This reorganization of the 2s and three 2p orbitals is called
hybridization. The four atomic orbitals on carbon (one 2s
and three 2p) hybridize and form four identical orbitals each 25
% 's' character and 75 % 'p' character. These new orbitals are
called sp3 hybrid orbitals. They are each singly occupied and can
bond with a 1s orbital on hydrogen forming four identical C-H
bonds. We already know the geometry of this molecule is
tetrahedral. The bond angle between each adjacent hybrid
orbital is 109.5 degrees.
The same hybridization can be used to describe the bent
geometry in H2O and the trigonal pyramidal geometry in
NH3. In water two of the sp3 hybrid
orbitals contain a lone pair of electrons. In NH3 one
of the hybrid orbitals contains a lone pair of electrons.