Chapter 10 Bonding Models
The bonding model we will focus on in this course is called Valence Bond Theory.
This theory of bonding considers the atomic orbitals on the atoms in a chemical
bond as overlapping to form a covalent bond. The easiest way to think about
VB Theory is to consider the atoms in a chemical bond as each contributing a
single electron to the covalent bond. In Valence Bond Theory we want to know
what atomic orbitals on each atom in the covalent bond overlap to form the covalent
bond. To determine that we need to look at the Lewis structure to know which
atoms are bonding, and then to consider the electron configuration of the neutral
atoms involved is the bond.
Let's consider some examples;
In hydrogen, H2, as the two hydrogen atoms approach one another
their spherical 1s orbitals begin to overlap.
Each electron occupying the space around the two nuclei. Each electron is attracted
simultaneously by each nuclei. The attraction that bonds the electrons to both
nuclei is the force holding the atoms together. Thus while ions do not exist
in covalent compounds the bond can be regarded as arising from the attraction
of oppositely charged particlesnuclei and electrons.
We can represent the formation of the covalent bond in hydrogen by writing
the Lewis electrondot formula for the atoms and the molecule.

The two electrons between the two hydrogen nuclei represent a covalent
bond. And the two electrons are referred to as an electron pair.
Each hydrogen atom can be thought of as sharing the pair of electrons. When
we think in these terms we note that each atom has a 1s2 electron
configuration, isoelectronic with the next noble gashelium.
The formation of the covalent bond in gaseous HF can be described in similar
terms;

The fluorine atom (1s22s22p5) and the hydrogen
atom (1s1) each require one electron to achieve a configuration isoelectronic
with a noble gas. Adding an electron to fluorine would make 10 electrons isoelectronic
with neon, adding an electron to hydrogen makes it isoelectronic to helium.
The two atoms share the electron pair to achieve their respective noble gas
configurations. In the fluorine atom the unpair electron is in a 2p orbital,
and in hydrogen the unparied electron is in a 1s orbital. We can draw pictures
of what the atomic orbitals look like on a fluorine atom and on a hydrogen atom.
When the covalent bond is formed between the fluorine and the hydrogen atoms
the 2p atomic orbital on fluorine overlaps with the 1s atomic orbital on hydrogen.
(Look at the Shockwave animation depicting this.) The
other three pairs of electrons around the fluorine atom are called nonbonding
or lone pair electrons.
If approach H2O, and NH3 in the same way we can determine
the atomic orbitals that overlap in those two cases also.
What atomic orbitals on the oxygen atom and the hydrogen in water overlap?
Answer
What atomic orbitals on the nitrogen atom and the hydrogen in ammonia overlap?
Answer
We can understand the formulas of a large number of covalent compounds by
writing or drawing the Lewis electrondot formulas for the compounds.
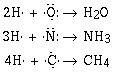
(Use lines to denote the covalent bond and dots to denote the electron
pairs.)
Here is a Shockwave file showing the Valence Bond model
applied to HF, H2O, and NH3.
When we look at the formula for the simplest compound containing carbon and
hydrogen it is found to be CH4. When we measure the C-H bond distances
they are all the same length and the H-C-H bond angles are all 109.5 degrees.
The electron configuration for carbon is 1s22s22p2.
The orbital diagram is;
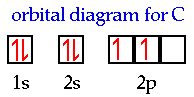
In the previous three examples the H-F, H-O and H-N bonds always
involved a 2p atomic orbital on the central atom and a 1s atomic orbital on
hydrogen to form the bond. How does that work in CH4? We see two
half-filled 2p atomic orbitals and one empty 2p atomic orbital...how do we get
four equivalent C-H bonds? There aren't four 2p atomic orbitals?
So now we have several problems that must be addressed;
1) The H-O-H bond angle in water is 105 degrees. But if we predicted
the bond angle in water based on the overlap of the atomic orbitals we would
say the H-O-H angle is 90 degrees. What's wrong with our prediction? Why is
the actual angle so different from the predicted?
2) The H-N-H bond angle in water is 107 degrees. But if we predicted
the bond angle in ammonia based on the overlap of the atomic orbitals we would
say the H-N-H angle is 90 degrees. What's wrong with our prediction? Why is
the actual angle so different from the predicted?
3) We see two half-filled 2p atomic orbitals and one empty 2p
atomic orbital...how do we get four equivalent C-H bonds? There aren't four
2p atomic orbitals?
A two time Nobel Prize winner, Linus Pauling (a hero of mine)
suggested that valence atomic orbitals in molecules are different than the atomic
orbitals on isolated atoms. Pauling proposed the atomic orbitals on an atom
could mix (hybridize) in certain combinations to produce new orbitals called
hybrid orbitals. These hybrid orbitals would have slightly different shapes
and orientations, and would overlap with atomic orbitals on another atom to
form covalent bonds.
In class we will look at the energy level diagram for beryllium,
boron and carbon and consider how hybridizing the atomic orbitals change their
shape and orientation.
So let's look at carbon again and see how forming hybrid orbitals
can help us understand what carbon forms four bonds. To begin with let's consider
the energy level diagram for a carbon atom. (here is an animation
showing the energy level diagram for a carbon atom.) The energy level is shown
below;
Our hydrid orbital model has us promoting one of the electrons
in the 2s orbital into the empty 2p orbital. (Here is an animation
showing the four atomic orbitals.)
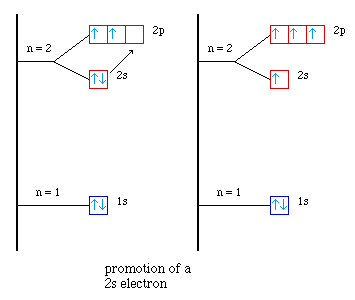
Then these four atomic orbitals are mixed together to form four
hybrid orbitals called sp3 hybrid orbitals. (Here is an animation
showing the hybrid orbital shape and orientation.)
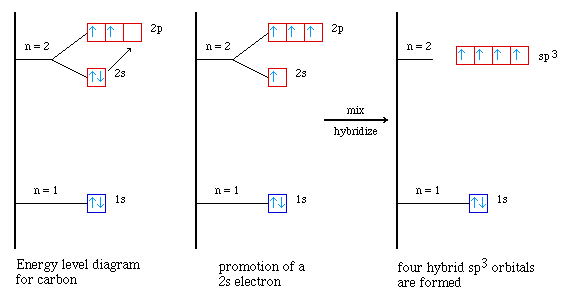
Here are the five animations we used in lecture.
When we consider methane, CH4, and draw a Lewis structure
for the molecule, the diagram we get has much more information in it.
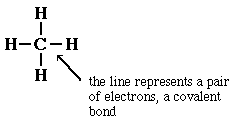
Now the line representing the covalent bond between the carbon
and the hydrogen takes on additional meaning. The line can be used to identify
the type of orbital on each atom involved in the chemical bond. In the case
of methane, the orbital on carbon is an sp3 hybrid orbital and the
orbital on hydrogen is the 1s orbital. These two orbitals, each with one electron,
overlap to product the covalent bond between carbon and hydrogen.
The bonding in both water, H2O and ammonia, NH3, can be described
in the same way. Although above I first introduced the bonding in water and
ammonia in terms of the atomic orbitals on oxygen (H2O) and on nitrogen (NH3)
we had a problem explaining the experimentally measured bond angles. In such
cases, conflicts between experimental evidence and a model, the model must be
adapted (or thrown away) to explain the experimental evidence. In this case
our inital model used atomic orbitals on oxygen and nitrogen to explain the
bonding in H2O and NH3. That model did not explain the bond angle. If we use
the hybrid model our correlation is much better. So for oxygen in water we could
imagine the same approach used with carbon in methane;
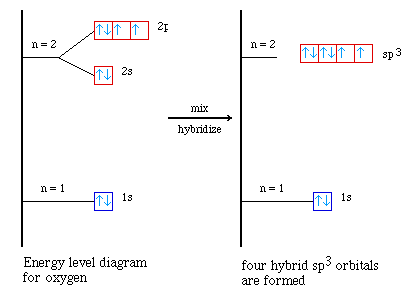
The minor difference is that we do not need to consider the 'promotion'
step as was needed in the case of carbon. Using sp3 hybrid orbital
on oxygen in water is much more useful in understanding the H-O-H bond angle.
Notice in the hybrized form there are four orbitals, two are filled (2 electrons)
and two are half-filled. The filled sp3 hybrid orbitals The ideal
bond angle for four groups of electrons around a central atom is 109.5 degrees.
For water the experimental bond angle is 104.5 degrees. The smaller angle can
be explained by the presences of the two lone-pairs of electrons on the oxygen
atom. Since they take up more volume of space compared to a bonding pair of
electrons the repulsions between lone pairs and bonding pairs is expected to
be greater causing the H-O-H bond angle to be smaller than the ideal 109.5 degrees.
We can imagine a similar diagram for nitrogen in ammonia.